Taking the Pulse of the Ross Ice Shelf
Ice Shelf Dynamics
On Antarctica, a massive continental ice sheet covers most of the land area and flows outward toward the coasts through numerous glaciers. Ice shelves are formed where flow rate is high and glaciers spill into somewhat protected waters, forming a long-lasting covering over the water below that can be several hundred meters thick. The Ross Ice Shelf is the largest of these in Antarctica. Unlike seasonal sea ice, ice shelves persist year-round and are significantly thicker, as seen in this photograph where the Ross Ice Shelf meets the seasonally ice-covered Ross Sea.
Buttressing
Even though the speed of ice flow on the Ross Ice Shelf is from 300-1000 meters per year, the mass of the floating ice shelf serves to buttress or restrain the flow of outlet glacier ice from land to the sea, keeping sea level rise in check. In recent years however, some Antarctic ice shelves have undergone rapid collapse, initiating large acceleration of glaciers into the ocean (Rignot et al., 2004; Scambos et al., 2004).
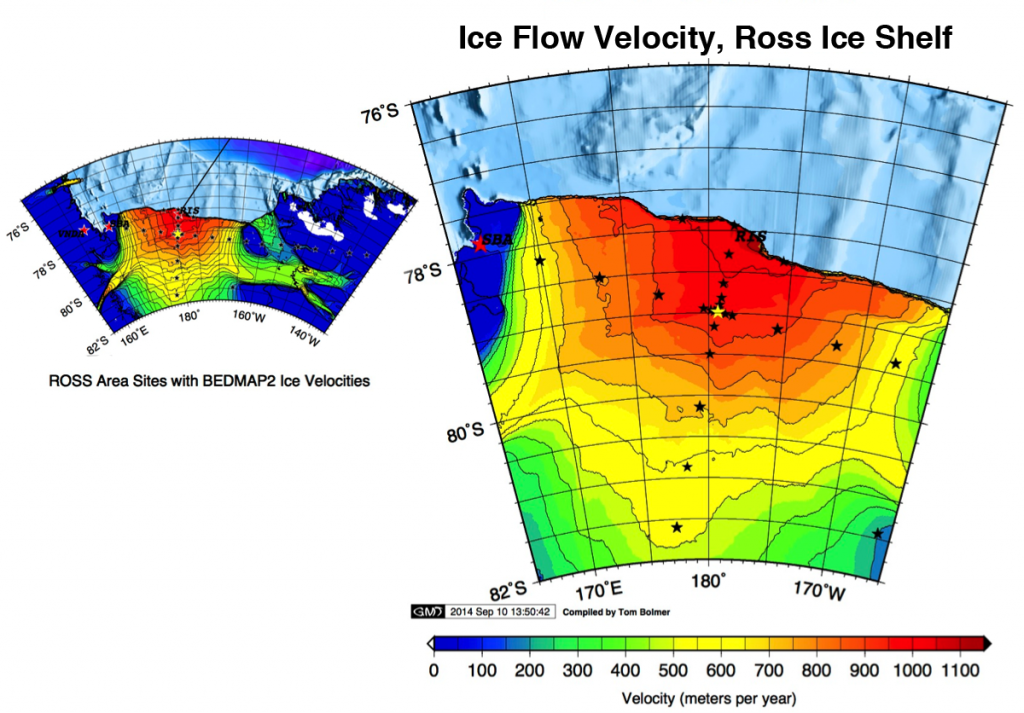
Ice flow velocity on the Ross Ice Shelf, Antarctica. The DRRIS seismometer array extends roughly north-south through the shelf center, from the shelf front to approximately 82°S.
The DRRIS seismometer array orthogonal to the ice front tracks a zone of high velocity flow. It is anticipated that thinner, faster portions of the RIS nearer the front should be less rigid and respond more strongly to flexural stresses induced by IG waves propagating in the water cavity below, with the amplitude of the response decreasing southward as the ice shelf thickens. These measurements will provide baseline measurements of the variability of RIS rigidity away from the front.
Catastrophic collapse
On the Antarctic Peninsula, a portion of the Larsen Ice Shelf larger than Rhode Island experienced such a collapse over the course of about 2 months in 2002.
Collapse of an ice shelf can occur when adjustment to environmental changes results in the thinning and weakening of the ice shelf, causing formation of large crevasses that increase the risk of the shelf breaking up into fragments. Bending stress from both the weight of the ice near the shelf front and flecture caused by wave and tide interactions, combined with hydrofractures from surface ice melt, are thought to contribute to this process. What forcing ultimately triggers a collapse event has not been determined, although ocean waves (swell and infragravity waves) are potential triggers.
References & Further Information
Bromirski, P.D., O.V. Sergienko, D.R. MacAyeal (2010). Transoceanic infragravity waves impacting Antarctic ice shelves, Geophys. Res. Lett., 37, L02502, doi:10.1029/2009GL041488.
MacAyeal, D.R., and 13 others (2006). Transoceanic wave propagation links iceberg calving margins of Antarctica with storms in tropics and Northern Hemisphere, Geophys. Res. Lett., 33(17), L17502.
Rignot, E.J., G. Casassa, P. Gogineni, W. Krabill, A. Rivera, and R. Thomas (2004). Accelerated ice discharge from the Antarctic Peninsula following the collapse of Larsen B ice shelf, Geophys. Res. Lett., 31, L18401, doi: 10.1029/2004GL020697
Scambos, T.A., J.A. Bohlander, C.A. Shuman and P. Skvarca (2004). Glacier acceleration and thinning after ice shelf collapse in the Larsen B embayment, Antarctica, Geophys. Res. Lett., 31(18), L18402 doi:10.1029/2004GL020670
Scambos, T., C. Hulbe, M. Fahnestock and J. Bohlander (2000). The link between climate warming and ice shelf break-ups in the Antarctic Peninsula, J. Glaciol., 46(154), 516-530.
Scambos, T., H.A. Fricker, C.-C. Liu, J. Bohlander, J. Fastook, A. Sargent, R. Massom and A.-M. Wu (2009). Ice shelf disintegration by plate bending and hydro-fracture: Satellite observations and model results of the 2008 Wilkins ice shelf break-ups, Earth Planet Sci. Lett., 280(1-4), 51-60.
Sergienko, O.V. (2010). Elastic response of floating glacier ice to impact of long‐period ocean waves. J. Geophys. Res., 115, F04028, doi:10.1029/2010JF001721.
Squire, V.A. (2007). Of ocean waves and sea ice revisited, Cold Reg. Sci. Technol., 49, 110-133.